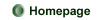

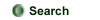
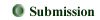


Original Paper
|
|
Acta Biochim
Biophys Sin 2009, 41: 677–688
|
doi: 10.1093/abbs/ gmp056.
|
Expression and function on embryonic development of lissencephaly-1 genes
in zebrafish
Chengfu Sun, Mafei Xu, Zhen
Xing, Zhili Wu, Yiping Li, Tsaiping Li, and Mujun Zhao*
State Key Laboratory of Molecular Biology, Institute of Biochemistry
and Cell Biology, Shanghai Institutes for
Biological Sciences,
Chinese Academy of
Sciences, Shanghai
200031, China
*Correspondence address. Tel: +86-21-54921115; Fax: +86-21-54921011; E-mail: [email protected]
Lissencephaly is a severe disease characterized by
brain malformation. The main causative gene of lissencephaly
is LIS1. Mutation or
deletion of LIS1 leads to proliferation and migration deficiency of
neurons in brain development. However, little is known about its biological
function in embryonic development. In this article, we identified the
expression patterns of zebrafish LIS1 gene and investigated
its function in embryonic development. We demonstrated that zebrafish
consisted of two LIS1 genes, LIS1a
and LIS1b. Bioinformatics
analysis revealed that LIS1 genes were conserved in evolution both in protein
sequences and genomic structures. The expression patterns of zebrafish LIS1a
and LIS1b showed that both
transcripts were ubiquitously expressed at all embryonic developmental stages
and in adult tissues examined. At the protein level, the LIS1 products mainly
exist in brain tissue and in embryos at early stages as shown by western
blotting analysis. The whole-mount immunostaining
data showed that LIS1 proteins were distributed all over the embryos from
1-cell stage to 5 day post-fertilization. Knockdown of LIS1 protein expression
through morpholino antisense oligonucleotides
resulted in many developmental deficiencies in zebrafish,
including brain malformation, circulation abnormality, and body curl. Taken
together, our study suggested that zebrafish LIS1 plays a very
important role in embryonic development.
Keywords LIS1; expression;
embryonic development; zebrafish
Received: March 17, 2009 Accepted: April 28, 2009
Introduction
Lissencephaly is a rare brain malformation disease caused by defects in neuronal
migration [1]. Classical lissencephaly has two major
types: Miller–Dieker syndrome (MDS) and
isolated lissencephaly sequence (ILS). All MDS and
some cases of ILS have haploinsufficiency at human
chromosome 17p13.3, from where LIS1 (Lissencephaly-1) gene was isolated [2]. Mutation or deletion of LIS1 leads to proliferation
and migration deficiency of neurons in brain development [3]. Thus, LIS1 is the main causative
gene responsible for classical lissencephaly.
Human LIS1, which
is highly expressed in brain, encodes a protein of 45 kDa
in size. LIS1 protein contains a Lis-H domain at its
N-terminus, followed by a coiled-coil region and seven WD repeats. LIS1
functions as a dimer in vivo. Lis-H domain
and the coiled-coil region are believed to be important for LIS1 dimerization, stability, and localization [4,5], while WD
repeats are required for protein interactions, through which LIS1 interacts
with a variety of proteins, such as tubulin [6], dynein [7], dynactin [7],
CLIP-170 [8], DCX [9], and NudeL [10], conferring its
influences on neuronal migration and mitotic cell division. LIS1 was also
identified as a subunit of platelet-activation factor (PAF) acetylhydrolase
[11], which regulates the physiological activity of PAF.
Although human LIS1 is a brain disease-caused gene, it is much conserved in evolution. The LIS1 gene has also been
identified in other species besides human, such as budding yeast [12], Aspergillus nidulans [13], nematode worm
[14], fruit fly [15], chick [16], and mouse [17]. Zebrafish
(Danio rerio) LIS1 genes have also been cloned, named LIS1a and LIS1b, and a
function of zebrafish LIS1 in
positioning cell nucleus in photoreceptors was found [18]. However, a basic
understanding of LIS1 genes is lacking in zebrafish. Because zebrafish has emerged as an important vertebrate model
system in development, owing to its unique advantages, such as production of
large amounts of transparent eggs and rapid and external embryonic development
[19], it will be interesting to investigate the function of LIS1 genes during embryonic
development in zebrafish. In this article, we
identified the LIS1 genes in zebrafish, analyzed their expression
patterns and investigated their functions on embryonic development. Our results
showed that both transcripts of zebrafish LIS1a and LIS1b were
expressed at all embryonic developmental stages and in adult tissues examined.
The LIS1 proteins were mainly detected in brain tissue and early developmental
embryos. The whole-mount immunostaining data showed
that LIS1 proteins were distributed all over the embryos from 1-cell stage to 5
day post-fertilization (dpf). Cellular localizations
of zebrafish LIS1 proteins were shown in the cell
cytoplasm. Knockdown of zebrafish LIS1 genes led to embryonic
developmental deficiency. Our results suggested that zebrafish
LIS1 genes are necessary
for embryonic development.
Materials and Methods
Zebrafish and cell lines
Zebrafish were provided by the Lab of Germ Cell and Embryonic Developmental Biology
in the Shanghai Institutes for Biological Sciences (Shanghai, China)
and maintained at 28.5ºC on a cycle of 14 h of light and 10 h of darkness. Fish breeding and
embryo manipulation were conducted according to established protocols [20].
Human HEK-293T and HeLa cells were obtained from the
Cell Bank of the Chinese Academy of Sciences (Shanghai, China)
and were maintained in our laboratory. These cell lines were cultured in
Dulbecco’s
modified Eagle’s medium (DMEM; Gibco
BRL, Grand Island, USA) supplemented with 10% (v/v) fetal bovine serum (FBS; Gibco BRL).
Extraction of RNA and RT–PCR
Total RNA was extracted from zebrafish embryos
and tissues using Trizol reagent (Invitrogen,
Carlsbad, USA) as described previously [21].
RNA preparation was digested with DNase I to
eliminate possible genomic DNA contamination. RT reactions were performed using
the SuperScript II RT–PCR kit (Gibco BRL) according to the
manufacturer’s
recommendations. To examine the expression patterns of zebrafish
LIS1a and LIS1b, RT–PCR was carried out to amplify the fragments of LIS1a (150 bp) and LIS1b (200 bp) with
primers listed in Table 1 (lisRT_F and lisRT_R).
PCR conditions were as follows: 94ºC for 2 min; 30 cycles of 94ºC for 20 s, 56ºC for 20 s, 72ºC for 20 s; 72ºC for a 5 min
extension. An 893-bp fragment of zebrafish b-actin (the sequence at GenBank under accession number
AF057040) was used as a control and amplified with primers actin_F
and actin_R (Table 1), under the conditions: 94ºC for 2 min; 30 cycles
of 94ºC for 30 s, 50ºC for 30 s, 72ºC for 1 min; 72ºC for a 5 min. PCR products were separated on 12% polyacrylamide
gel for LIS1a and LIS1b followed with silver
staining, or 1% agarose gel for b-actin and stained with Goldview (SBS, Beijing, China).
Plasmid construction
To generate plasmids pEGFP-C1-LIS1a and pEGFP-C1-LIS1b, the full length of coding
sequences of zebrafish LIS1a (GenBank accession No. NM_201346) and LIS1b (GenBank
accession No. NM_201345) were amplified from zebrafish
liver cDNA using PyroBest
polymerase (TaKaRa, Dalian, China).
Touchdown PCR was used to clone zebrafish LIS1a with primers lis1a_EF
and lis1a_XR (Table 1) and cycling
condition was an initial step of 94ºC for 2 min, followed by 10 cycles of 94ºC for 30 s, annealing
temperatures starting at 60ºC for 30 s, decreasing by 0.5ºC per cycle, and 72ºC for 90 s for
extension. This step was followed by 25 cycles of 94ºC for 30 s, 55ºC for 30 s, and 72ºC for 90 s; and a
final extension at 72ºC for 5 min. For cloning of LIS1b, primers lis1b_F and lis1b_R (Table 1) were used with first cycling conditions: a 2 min initial denaturing step
at 94ºC; followed by 30 cycles of 94ºC for 30 s, 53ºC for 30 s, and 72ºC for 90 s; and a
final extension step at 72ºC for 5 min. PCR product was used as the
template for a second round PCR with primers lis1b_EF and lis1b_XR (Table 1) and the cycling
condition was same as cloning of LIS1a. The PCR fragments of
LIS1a and LIS1b were cut
with EcoRI and XhoI and inserted into pEGFP-C1 vector (Clontech, Mountain
View, USA).
The two plasmids were used for subcellular
localization analysis.
Plasmid pCS2-lismo-GFP was generated by PCR amplifying of the 5'-UTR sequences of zebrafish
LIS1b with primers listed in
Table 1 (lismo_BF
and lismo_ER) and inserted into pCS2-GFP (kept in our
Lab). PCR was performed with the same cycling conditions as cloning of LIS1a with Taq polymerase from BioDev (Beijing, China).
The PCR fragment was ligated to pMD-18T (TaKaRa), cut with BamHI and EcoRI and inserted into
pCS2-GFP. The plasmid was used for RNA transcription and analysis of morpholino efficiency.
Transient transfection and subcellular localization
Cells were transiently transfected with pEGFP-C1
vector or pEGFP-C1-LIS1 plasmids. Transfections were
performed with Lipofectamine 2000 (Invitrogen) according to the manufacturer’s instructions. Briefly,
HEK-293T or HeLa cells were seeded into 24-well
plates, and 0.4 mg of plasmid was mixed with 0.6 ml transfection
agent per well and added to cells. After 24 h transfection,
HEK-293T cells were collected for western blotting analysis; HeLa cells were washed three times with PBS and then fixed
for 10 min in chilled methanol. The nuclei were stained with Hoechst 33258 for
5 min. After several washes, coverslips were sealed
and the cells were examined under a fluorescence microscope (Olympus BX51;
Olympus, Tokyo, Japan).
Preparation of antibody
Rabbit polyclonal antibody against the N-terminus of human LIS1 peptide
(residue 1–55 amino acids) fused to N-terminal GST tag was prepared in our
laboratory. The specificity of the antibody was examined by western blotting
analysis.
Protein extraction and western blotting analysis
For the preparation of proteins from embryos, zebrafish
embryos of different developmental stages were dechorionated
and cells were scattered by pipette aspiration. After centrifuged at 6000 g for 1 min, supernatants were
removed and cell pellets were dissolved in sodium dodecyl
sulfate (SDS) sample buffer (50 mM Tris–HCl, pH 6.8, 100 mM DTT, 2% SDS, 20%
glycerol, and 0.2 mg/ml bromophenol blue). For the
preparation of proteins from tissues, zebrafish
tissue samples were homogenized in liquid nitrogen, lysed
with RIPA buffer (20 mM
Tris–HCl, pH 7.5, 1% Triton
X-100, 150 mM
NaCl, 2 mM EDTA, 10% glycerol, 10 mM NaF, and 1 mM Na3VO4) containing Protease Inhibitor
Cocktail (P8340; Sigma, St. Louis, USA) on ice for 30 min and centrifuged at 16,000 g
for 20 min at 4ºC. Supernatant fractions were collected and dissolved in appropriate SDS
sample buffer. For western blotting analysis, the proteins were transferred
onto nitrocellulose membranes (Amersham Pharmacia, San Francisco, USA)
and immunoblotted with the following primary
antibodies: anti-LIS1 antibody (dilution 1:2000) or antitubulin
mAb (dilution 1:500; A54 Cocktail NeoMarkers,
Fremont, USA). After washing, the membranes
were incubated with goat anti-rabbit or mouse IgG-HRP
secondary antibodies (dilution 1:2000; Santa Cruz Biotechnology, Santa Cruz, USA).
Immunodetection was performed by the luminescence
method using ECL reagents kit (Perfect Biotech, Shanghai, China),
and the membranes were then exposed to an X-Omat
film.
Whole-mount immunochemistry
Whole-mount immunochemistry was performed according to Hashimoto et al. [22]. Briefly,
embryos of different developmental stages were fixed for 24 h with 4% paraformaldehyde at 4ºC, dechorionated
and washed with PBST (PBS with 1% Tween-20), and blocked with blocking buffer
(PBST containing 10% heat inactivated goat serum). Preimmune
serum or anti-LIS1 antibody (dilution 1:300 in PBST containing 1% goat serum) was added, and
the embryos were incubated overnight at 4ºC. After washing three
times for 30 min each, the embryos were incubated with fluorescein
isothiocyanateconjugated rat anti-rabbit IgG (dilution 1:100; 111-096-144; Jackson Immunoresearch, West
Grove, USA) for 10 h at 4ºC. After washing three
times for 15 min each, the specimens were examined using a fluorescence
stereoscopic microscope (DP71; Olympus).
Capped RNA transcription, morpholino
oligonucleotides, and microinjection
To produce capped lismo-GFP RNA, the
pCS2-lismo-GFP plasmid was linearized by restriction
enzyme NotI, followed by in vitro transcription using the SP6 mMessage mMachine kit (Ambion, Austin,
USA).
Antisense morpholino oligonucleotides
(MOs) against zebrafish LIS1a (MO-LIS1a-ATG: 5'-CTCGTTGCCTCTGTGACAGCACCAT-3', sequence was
provided by Tsujikawa [18]), LIS1b (MO-LIS1b1: 5'-ACGTTGCCTCTGTGACAGCACCATG-3'; MO-LIS1b2: 5'-TCAGGTGTAGAGTGTGCAGCAACTG- 3', which were designed by Gene Tools, LLC), and
a standard MO control (MO-Sdc: 5'-CCTCTTACCTCAGTTACAATTTATA-3') were synthesized by
Gene Tools, LLC (Philomath, USA).
The antisense MOs were microinjected into the yolk of 1- or 2-cell embryos. The
lismo-GFP RNA (100 ng/ml) and MOs (4 ng/nl) were injected in embryos with the injection volume
of 4 nl per embryo. Phenol red (0.05%) was
co-injected as a non-toxic injector tracer. Embryos were photographed with a
digital camera (DP71; Olympus).
Results
Bioinformatics analysis of zebrafish
LIS1 genes
By GenBank searching, we found that LIS1 homologous genes
existed in 29 species including 18 vertebrates and 11 invertebrates. To infer
evolutionary relationships of zebrafish LIS1 homologues, we
constructed a phylogenetic tree based on the protein
sequences of LIS1 from these 29 species using the neighbor-joining method (Fig. 1). The phylogenetic tree showed that zebrafish
LIS1 (D. rerio-a and D. rerio-b) were evolutionarily
conserved, especially among the vertebrate species. It is interesting that the
similarity of LIS1 proteins between social ameba and human, the positions of
which are very distant in evolution, is still as high as about 64% identical,
suggesting that LIS1 gene appeared very early in evolution and evolved in a much conserved way.
To analyze the relatedness between the zebrafish LIS1
proteins and other animal model species more detailedly,
multiple amino acid sequence alignments of the human, mouse, zebrafish, fruit fly, nematode worm, and yeast were
performed with ClustalX software (ftp:// ftp-igbmc.u-strasbg.fr/pub/ClustalX). Results showed that LIS1
proteins including LIS1a
and LIS1b are more conserved in evolution [Fig. 2(A)]. Both LIS1a and LIS1b consist of three motifs: N-terminal Lis-H domain followed by a coiled-coil region and seven WD
repeats. All of these motifs are highly conserved among different species. The
lengths of different LIS1 proteins are also very similar except yeast, which is
a little longer. The percentages of sequence identity among different species
were also calculated [Fig. 2(B)], which showed that zebrafish LIS1 proteins
have high identities with human and mouse (94% of LIS1a and 95% of LIS1b). LIS1b is a little more conserved
than LIS1a. Zebrafish contains two LIS1 genes: LIS1a and LIS1b, which
is different from other species. They are located on different chromosomes with
LIS1a on chromosome 21 and LIS1b on chromosome 15. In order to understand the relationship of zebrafish and mammalian LIS1 genes in vertebrate genome evolution, syntenic analysis was performed between human and zebrafish. Human LIS1 was used as the anchor site; orthologous
comparisons of the genes in the regions flanking the LIS1 loci of human and zebrafish
genomes were performed with NCBI-BLAST. Result showed that more genes in the
vicinity of human LIS1 were mapped to the neighborhood of zebrafish LIS1b locus than LIS1a locus [Fig. 2(C)]. This suggests that LIS1b may be evolved to mammalian LIS1 conservatively, while zebrafish LIS1a is diverged more rapidly and may be lost during evolution.
Expression patterns of zebrafish
LIS1 transcripts
Alignment of LIS1a
and LIS1b sequences showed that
there were some gaps in the 3'-UTR of LIS1a sequence [Fig. 3(A)]. To examine the expression of zebrafish LIS1a and LIS1b transcripts,
we designed specific primers (lisRT_F and lisRT_R) to amplify sequences covering this region to
produce the 150 bp fragment of zebrafish
LIS1a and 200 bp fragment of LIS1b. We then analyzed the expressions of zebrafish LIS1a
and LIS1b mRNA by RT–PCR at different embryonic developmental stages. We found that both zebrafish LIS1a
and LIS1b were ubiquitously
expressed at all developmental stages including 2 h post-fertilization (hpf ) (64-cell), 10 hpf (tail
bud), 24 hpf ( prim-5), 48 hpf
(Long pectoral fin bud), 72 hpf (Protruding mouth),
and 120 hpf (swimming larva) [Fig. 3(B)]. The existence of zebrafish LIS1a
and LIS1b in the 2 hpf embryonic cleavage stage, when the embryonic genome is transcriptionally inactive [23], indicates that zebrafish LIS1 messages are maternal RNA stored in the eggs. We also analyzed the
expressions of zebrafish LIS1a and LIS1b in
different zebrafish adult tissues including heart,
brain, liver, intestine, muscle, spleen, ovary, eye, gill, fin, and testis. As
shown in Fig. 3(C), zebrafish LIS1a and LIS1b were also
ubiquitously expressed in all eleven tissues examined. The concordant
expression of zebrafish LIS1a and LIS1b during
embryonic development and in adult tissues suggests that the two genes may be
controlled by similar mechanisms of transcriptional regulation.
Expression patterns of zebrafish
LIS1 proteins
To examine the expression of zebrafish LIS1
proteins at different embryonic developmental stages and adult tissues, western
blotting analysis with polyclonal antibody against human LIS1 was used. The
efficiency of the antibody against zebrafish LIS1
protein was examined. It showed that the antibody could recognize exogenous zebrafish LIS1a
and LIS1b proteins [Fig. 3(D)]. Then we assayed the expression of LIS1 proteins in embryos. The zebrafish LIS1 proteins were mainly expressed in 6, 10, and
24 hpf embryos, but not detectable in 2 hpf, and with very low expression after 72 hpf [Fig. 3(E)]. We next analyzed the zebrafish LIS1 proteins
in adult tissues such as eye, brain, liver, gill, fin, ovary, and testis. As
shown in Fig. 3(F), zebrafish LIS1 proteins were expressed at high
levels in brain tissue, but not detectable in other six tissues. As the zebrafish LIS1a
and LIS1b mRNA could be detected
in all tissues examined by RT–PCR, we interpret that
the absence of LIS1 proteins in tissues may result from the low sensitivity of
polyclonal antibody which might not be able to recognize the very low levels of
zebrafish LIS1 proteins in the tissues.
Whole-mount immunostaining was also used to
investigate the expression patterns of LIS1 proteins during embryogenesis. The
results indicated that zebrafish LIS1 proteins were
distributed all over the embryos from 1-cell stage to 5 dpf,
as well as in the unfertilized eggs (Fig. 4). In the unfertilized eggs [Fig. 4(A–D)], LIS1 proteins were
distributed evenly in the eggs [Fig. 4(B)]; with the advance of cytoplasmic streaming,
cytoplasm was separated from yolk, LIS1 proteins were translocated
to the putative animal pole, and accumulated there gradually [Fig. 4(C,D)]. After
fertilization, LIS1 proteins were accumulated at the animal pole during the
1-cell stage as in unfertilized eggs and then distributed throughout the blastomeres in the cleavage period [Fig. 4(F–H)]. Intensity of LIS1 expressions was much higher in fertilized embryos
than unfertilized eggs. At the 4-cell stage, we found that LIS1 proteins were
not evenly distributed, with lower expression in some areas of blastomeres [Fig. 4(G)]. Generalized distribution of LIS1 proteins persisted from shield stage
to 5-somite stage; it was disturbed after 24 hpf [Fig. 4(I,J,M)]. From 24 hpf to 5 dpf, the LIS1 proteins
were highly expressed in brain than other tissues [Fig. 4(K,L,O)], suggesting that LIS1 proteins are very
important for brain development.
Subcellular localizations of zebrafish
LIS1 proteins
To identify the subcellular localizations of zebrafish LIS1 proteins, we constructed the GFP-fused LIS1a and LIS1b expression plasmids. After transfection of human HeLa cells
with pEGFP-C1 control vector, pEGFP-C1-LIS1a, or pEGFP-C1-LIS1b plasmid, cellular distributions
of GFP-LIS1 proteins were examined under the fluorescence microscope. We found
that zebrafish GFP-LIS1a and GFP-LIS1b were both localized in the cell
cytoplasm, but none in cell nucleus, comparing with the GFP control (Fig. 5). The data
demonstrated that the localizations of zebrafish LIS1
proteins were mainly in the cell cytoplasm, which were the same as that of
human and mouse LIS1 proteins [7,10,24].
Knockdown of zebrafish LIS1
proteins affects embryonic development
Owing to the advantages of zebrafish over other
animal system, especially the external embryonic development, we examined the
effect of LIS1 on zebrafish embryonic development using antisense MO
technology. Three MOs (MO-LIS1a-ATG,
MO-LIS1b1, and MO-LIS1b2) were used to
knockdown the expression of endogenous LIS1 proteins. The MO-LIS1a-ATG, against LIS1a, had been determined to inhibit LIS1a protein expression efficiently [18]. The efficiency
of MO-LIS1b1 and MO-LIS1b2 corresponding to the 5'-UTR and the beginning of ORF of the LIS1b gene were determined.
The lismo-GFP RNA mixed with different MOs tested
were injected into embryos. Results showed that the expression of GFP protein
was inhibited in embryos injected with the mixture of lismo-GFP
and MO-LIS1b1 or lismo-GFP
and MO-LIS1b2, while in embryos
injected with lismo-GFP and MO-Sdc control, the
expression of GFP was not inhibited, the same as lismo-GFP
only [Fig. 6(A)]. This result demonstrated that the two designed LIS1b morpholinos could efficiently
inhibit the expression of LIS1b. To examine the phenotype changes of embryos
after injection of MO-LIS1, six groups of MOs including individuals and combinations of MO-LIS1a-ATG, MO-LIS1b1, and MO-LIS1b2 as listed in Table 2, were injected into 1- or 2-cell embryos. We found that, compared with
the control MO group, the embryos after injection of MO-LIS1 displayed many developmental defects. The abnormal phenotypes can be classified
into three levels: slight, moderate, and severe degrees. The pictures showing
the MO-LIS1a-ATG morphants represented the
typical developmental deficient embryos at three degrees [Fig. 6(B,D)]. The embryo defects
could be observed at 12 hpf with developmental delay
of presumptive brain and tail bud. Midbrain-hindbrain boundary (MHB) [25],
which organizes the formation of the midbrain and cerebellum, was disrupted. At
30 hpf, brain structures, including optic vesicles,
were developed irregularly possibly with cell necrosis. Heartbeat was delayed
and embryos moved stiffly. At 60 hpf, embryos
developed small eyes, thin pigmentation, and abnormal circulation. The
comparison of phenotypes between normal embryos and MO-LIS1a-ATG
morphants at same stages was indicated [Fig. 6(C)]. The borders of optic vesicles and notochord were blurry at 16 hpf morphants [Fig. 6(C), a']. The irregular brain structures with cell
necrosis appeared at 30 hpf morphants
[Fig. 6(C), b']. The small eyes and head, thin pigmentation,
abnormal circulation, and disorganized musculature were observed at 5 dpf morphants [Fig. 6(C), c' and d']. Most embryos (88%, n = 16) displayed curled body as raf1 morphant [26] and other deficiencies, and died
within 9 days. We also found that injection of morpholino
combinations displayed much severe embryonic deficiencies, especially MO-LIS1a-ATG plus MO-LIS1b2 (Table 2). The ratios of abnormalities of different MO-LIS1 morphants were also shown in Table 2. Our results clearly
indicate that LIS1 genes are vital for zebrafish embryonic
development.
Discussion
LIS1 gene has been cloned in a variety of animals, including zebrafish, which was reported by Tsujikawa
et al. [18], while we had
also cloned zebrafish LIS1 genes. Interestingly, zebrafish
contains two LIS1 genes, LIS1a
(also called
pafah1b1b) and LIS1b (also called pafah1b1a),
which is different from other species, such as human, mouse, worm, fruit fly,
and yeast. We performed bioinformatics analysis to explore the evolution status
of LIS1 gene, and found that
it was more conserved in evolution, especially in vertebrates. The origination
of LIS1a and LIS1b appears
to be the result of gene or genome duplication in fish evolution [27], as we
found that the form of two LIS1 was also existed in Salmo salar (LIS1a, GenBank accession No. BT045765; LIS1b, GenBank
accession No. BT045543). Although zebrafish LIS1a and LIS1b share
much similarity in gene and protein sequences, we guess the evolutionary
consequences of them may be different. The sequence analysis [Fig. 1(C)] and gene synteny analysis [Fig. 1(D)] suggest that LIS1b may be contributed to evolve to mammalian, while LIS1a may be degenerated in evolution, since the identity of LIS1b is a little closer to
mammalian LIS1 than LIS1a. We also analyzed the genomic structures of zebrafish
LIS1 genes and compared
them with that of human LIS1. It showed that the genomic structure of zebrafish
and human is much alike with same numbers and similar sizes of exons and introns, indicating the
unique signature of zebrafish LIS1 genes in evolution.
To investigate the expression of LIS1a
and LIS1b, we designed specific
primers to amplify the transcripts for LIS1a and LIS1b. We
found that zebrafish LIS1a and LIS1b were
expressed with similar patterns, they are ubiquitously expressed in all
embryonic developmental stages and adult tissues examined. However, because of
the high similarity of these two proteins, it is difficult to prepare the
antibody that can distinguish between the LIS1a and LIS1b. Here, an anti-human LIS1 antibody was
used to identify the zebrafish LIS1 proteins, since
we had determined that human LIS1 antibody can recognize the zebrafish LIS1 proteins produced by 293T cells [Fig. 3(D)]. The western
blotting analysis showed that zebrafish LIS1 proteins
were highly expressed in brain tissue, but not in other tissues such as eye and
liver. Thus, the expression pattern of LIS1 at the protein level is different from that
at the mRNA level, which maybe resulted from the methodological sensitivity.
For example, with immunostaining method, zebrafish LIS1 proteins were shown at all embryonic
developmental stages from 1-cell to 5 dpf (Fig. 4), while using western
blotting LIS1 proteins only existed at embryos from 6 to 24 hpf
[Fig. 3(E)]. However, concerning
the tissue distribution of LIS1 proteins, the higher expression of LIS1
proteins in brain reflects the most important role of LIS1 in brain development. Consistent with our
results, the expression of LIS1 in
chicken also showed a ubiquitous expression in embryos of different
developmental stages, and a higher expression in brain than other tissues [16].
The subcellular localizations of zebrafish
LIS1a and LIS1b were shown in the
cytoplasm (Fig. 5), which
is the same as mammalian counterparts [7,10,24]. It implies that zebrafish LIS1 proteins may have similar cellular functions
as human LIS1, such as neuronal migration and mitotic cell division.
Studies in other animal systems suggest that LIS1 plays an important role in reproduction.
For example, depletion of LIS1 in nematode
worm led to embryo lethality and sterility [14]. Mutations of LIS1 in fruit fly resulted in partial ventralization of the eggshell and abnormal oocyte differentiation [15,28]. Knockout of LIS1 in mouse led to impairment in spermatogenesis
and embryo lethality [3,29]. In addition, inactivation of a testis-specific LIS1
transcript caused male
infertility [30]. However, detailed investigation of LIS1 in embryonic
development is lacking. Thus, we explored the function of zebrafish
LIS1 in embryonic
development due to the unique advantages of zebrafish.
After knockdown of the endogenous zebrafish LIS1
proteins with antisense MOs, zebrafish embryos
displayed many developmental deficiencies, such as growth delay, body curl,
brain malformation, small eyes, and circulation abnormality. Percentages of
abnormal phenotype after injection of LIS1a and LIS1b morpholinos mixtures (LIS1a-ATG
+ LIS1b1 and LIS1a-ATG + LIS1b2) are higher than that of single morpholinos.
This suggests that LIS1a
and LIS1b may corporate to execute their functions in embryonic development.
The developmental defects of zebrafish embryos may
reflect the important functions of LIS1 proteins in the cellular content, such
as active mitosis division and dynamic cell migration. Further investigations
may be needed to find out the functions and molecular mechanisms of zebrafish LIS1a
and LIS1b involved in embryonic development extensively.
Funding
This work was supported by grants from National Key S&T Special
Project of China (No. 2008ZX10002-020), and the Shanghai Municipal Commission
for Science and Technology (No. 74319111 and 07DZ22919).
References
1 Kato M and Dobyns WB. Lissencephaly
and the molecular basis of neuronal migration. Hum Mol Genet 2003, 1: R89–R96.
2 Reiner O, Carrozzo R, Shen
Y, Wehnert M, Faustinella
F, Dobyns WB and Caskey CT,
et al. Isolation of a
Miller–Dieker lissencephaly
gene containing G protein b-subunit-like repeats. Nature 1993, 364:
717–721.
3 Hirotsune S, Fleck MW, Gambello
MJ, Bix GJ, Chen A, Clark GD and Ledbetter DH, et al. Graded reduction of
Pafah1b1 (LIS1) activity results in neuronal migration defects and early
embryonic lethality. Nat Genet 1998, 19: 333–339.
4 Cahana A, Escamez T,
Nowakowski RS, Hayes NL, Giacobini
M, von Holst A and Shmueli
O, et al. Targeted mutagenesis
of LIS1 disrupts cortical development and LIS1 homodimerization.
Proc Natl Acad Sci USA 2001, 98: 6429–6434.
5 Gerlitz G, Darhin E,
Giorgio G, Franco B and Reiner O. Novel functional features of the Lis-H domain: role in protein dimerization,
halflife and cellular localization. Cell Cycle 2005,
4: 1632–1640.
6 Sapir T, Elbaum M and Reiner O. Reduction of
microtubule catastrophe events by LIS1, platelet-activating factor acetylhydrolase subunit. EMBO J 1997, 16: 6977–6984.
7 Smith DS, Niethammer M, Ayala R, Zhou Y, Gambello MJ, Wynshaw-Boris A and
Tsai LH. Regulation of cytoplasmic dynein behavior and microtubule organization by mammalian
LIS1. Nat Cell Biol 2000, 2: 767–775.
8 Tai CY, Dujardin DL, Faulkner NE and Vallee RB. Role of dynein, dynactin, and CLIP-170 interactions in LIS1 kinetochore function. J Cell Biol
2002, 156: 959–968.
9 Caspi M, Atlas R, Kantor A, Sapir T and Reiner
O. Interaction between LIS1 and doublecortin, two lissencephaly gene products. Hum Mol Genet 2000, 9: 2205–2213.
10 Sasaki S, Shionoya A, Ishida M, Gambello MJ, Yingling J, Wynshaw-Boris A and Hirotsune S.
A LIS1/NUDEL/cytoplasmic dynein heavy chain complex
in the developing and adult nervous system. Neuron 2000, 28: 681–696.
11 Hattori M, Adachi H, Tsujimoto M, Arai H and
Inoue K. Miller– Dieker
lissencephaly gene encodes a subunit of brain
platelet-activating factor acetylhydrolase. Nature
1994, 370: 216–218.
12 Fujiwara T, Tanaka K, Inoue E, Kikyo M and Takai Y. Bni1p regulates microtubule-dependent nuclear
migration through the actin cytoskeleton in Saccharomyces cerevisiae. Mol Cell Biol 1999, 19: 8016–8027.
13 Xiang X, Osmani AH, Osmani
SA, Xin M and Morris NR. NudF,
a nuclear migration gene in Aspergillus nidulans, is similar to the human LIS-1 gene required for neuronal migration. Mol Biol Cell 1995, 6: 297–310.
14 Dawe AL, Caldwell KA, Harris PM, Morris NR
and Caldwell GA. Evolutionarily conserved nuclear migration genes required for
early embryonic development in Caenorhabditis elegans. Dev Genes Evol 2001, 211: 434–441.
15 Liu Z, Xie T and Steward R. LIS1, the Drosophila homolog of a human lissencephaly disease gene, is required for germline cell division and oocyte
differentiation. Development 1999, 126: 4477–4488.
16 Shmueli O and Reiner O. Expression of chLIS1,
a chicken homolog of LIS1. Dev Genes Evol 2000, 210:
51–54.
17 Reiner O, Albrecht U, Gordon M, Chianese KA,
Wong C, Gal-Gerber O and Sapir T, et al. Lissencephaly gene (LIS1) expression in the CNS suggests a role in
neuronal migration. J Neurosci 1995, 15: 3730–3738.
18 Tsujikawa M, Omori Y, Biyanwila
J and Malicki J. Mechanism of positioning the cell
nucleus in vertebrate photoreceptors. Proc Natl Acad Sci USA 2007, 104: 14819–14824.
19 Kimmel CB. Genetics and early development of zebrafish.
Trends Genet 1989, 5: 283–288.
20 Westerfield M. The Zebrafish
book. A Guide for the Laboratory use of Zebrafish (Danio rerio). 4th edn. Eugene:
University
of Oregon
Press, 2000.
21 Sun C, Wu Z, Jia F, Wang Y, Li T and Zhao M.
Identification of zebrafish LPTS: a gene with
similarities to human LPTS/PinX1 that inhibits telomerase activity. Gene 2008,
420: 90–98.
22 Hashimoto Y, Suzuki H, Kageyama Y, Yasuda K
and Inoue K. Bruno-like protein is localized to zebrafish
germ plasm during the early cleavage stages. Gene Expr Patterns 2006, 6: 201–205.
23 Kane DA and Kimmel CB. The zebrafish midblastula transition. Development 1993, 119: 447–456.
24 Kondratova AA, Neznanov
N, Kondratov RV and Gudkov
AV. Poliovirus protein 3A
binds and inactivates LIS1, causing block of membrane protein trafficking and
deregulation of cell division. Cell Cycle 2005, 4: 1403–1410.
25 Rhinn M and Brand M. The midbrain–hindbrain boundary organizer. Curr Opin Neurobiol 2001, 11: 34–42.
26 Razzaque MA, Nishizawa
T, Komoike Y, Yagi H, Furutani M, Amo R and Kamisago M, et al. Germline gain-of-function mutations in RAF1
cause Noonan syndrome. Nat Genet 2007, 39: 1013–1017.
27 Meyer A and Schartl M. Gene and genome
duplications in vertebrates: the one-to-four (-to-eight in fish) rule and the
evolution of novel gene functions. Curr Opin Cell Biol 1999, 11: 699–704.
28 Lei Y and Warrior R. The Drosophila Lissencephaly1 (DLIS1) gene is required for nuclear migration. Dev Biol
2000, 226: 57–72.
29 Koizumi H, Yamaguchi N, Hattori M, Ishikawa TO, Aoki J, Taketo MM and
Inoue K, et al.
Targeted disruption of intracellular type I platelet activating factor-acetylhydrolase catalytic subunits causes severe impairment
in spermatogenesis. J Biol Chem
2003, 278: 12489–11294.
30 Nayernia K, Vauti
F, Meinhardt A, Cadenas C, Schweyer S, Meyer BI and Schwandt
I, et al. Inactivation of a
testis-specific LIS1 transcript in mice prevents spermatid
differentiation and causes male infertility. J Biol Chem 2003, 278: 48377–48385.